Crystal science encompasses a wide range of fascinating topics related to the study of crystals and crystalline materials. From determining crystal structures to analyzing crystal defects, crystal science provides key insights into the atomic and molecular arrangements responsible for a crystal’s macroscopic physical properties.
In this beginner’s guide, we’ll explore some of the main areas of crystal science to give you an introduction to this multifaceted field.
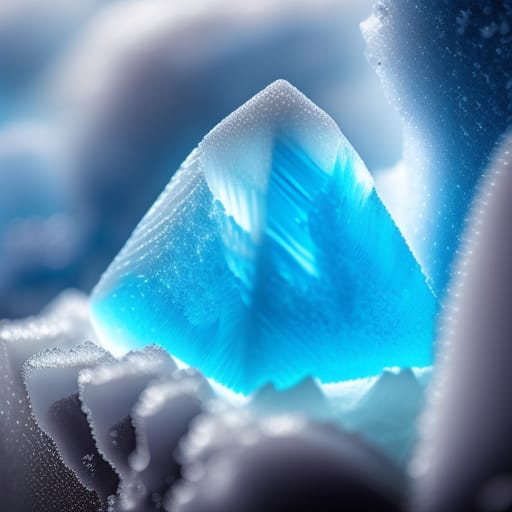
What is Crystal Science?
Crystal science is the broad study of the structure, properties, growth, defects, and applications of crystalline materials. Crystalline materials are solids made up of atoms, molecules, or ions arranged in an orderly, repeating 3D pattern known as a crystal lattice. This highly organized structure is what gives crystals their distinctive geometric shapes and physical characteristics.
Some of the main disciplines within crystal science include:
- Crystallography: The study of crystal structure and symmetry
- Mineralogy: The study of mineral composition and classification
- Crystal growth: The study of mechanisms and techniques for crystal growth
- Crystal defects: The study of imperfections in crystal structure
- Crystal optics: The study of light propagation in crystals
- Crystal engineering: The design and synthesis of crystalline materials
Understanding crystals is important across many fields including materials science, chemistry, physics, biology, and engineering. Crystal science provides insights that enable advances in semiconductor technology, pharmaceutical development, metallurgy, optics, and more.
Now let’s take a closer look at some of the key areas of crystal science.
Exploring Crystallography
Crystallography is the foundational discipline within crystal science concerned with understanding crystal structure and symmetry. Some of the main topics in crystallography include:
Crystal Systems
Crystals can be categorized into seven distinct crystal systems based on their symmetry and unit cell parameters:
- Cubic
- Tetragonal
- Orthorhombic
- Hexagonal
- Trigonal
- Monoclinic
- Triclinic
The cubic system is the most symmetric, while the triclinic system is the least symmetric.
Space Groups
Space groups describe the combination of translation symmetries and rotational/reflection symmetries present in a crystal structure. There are 230 possible space groups. Identifying a crystal’s space group is key to determining its properties.
Miller Indices
Miller indices are a notation system used to describe crystallographic planes and directions in three dimensions. The notation (hkl) defines a family of parallel planes intersecting the x, y, and z crystal axes.
X-Ray Diffraction
X-ray diffraction is an instrumental technique used to study crystal structure by diffracting X-rays off the electron clouds of the atoms. It reveals information about atomic arrangements and bonds.
Exploring Mineralogy
Mineralogy is the branch of crystal science concerned with the chemical composition, crystallographic properties, and classification of minerals. Some key aspects of mineralogy include:
Mineral Composition
Minerals are crystalline compounds formed from different elements. Major rock-forming mineral groups include silicates, carbonates, oxides, sulfides, and halides.
Mineral Structure
The crystal structure of a mineral is defined by its repeating atomic arrangement or lattice. Common structures include cubic, hexagonal, monoclinic, and triclinic.
Mineral Identification
Key properties used to identify minerals include cleavage, fracture, hardness, luster, color, streak, crystal habit, and density.
Mineral Classification
Minerals are classified based on their chemical composition and dominant anion group, such as halides, sulfides, oxides, carbonates, phosphates, and silicates.
Probing Crystal Growth
Another vital area of crystal science is crystal growth – the study of the thermodynamic and kinetic processes that occur when crystalline solids form from liquids, gases, or melts.
Growth Mechanisms
Key mechanisms of crystal growth include unit cell replication, adsorption layer growth, spiral growth, and polyhedral layer growth. These involve adding new atoms, molecules, or ions to the crystal lattice in an orderly way.
Growth Techniques
Common techniques to grow crystals in the lab include solution crystallization, melt growth, chemical vapor deposition, molecular beam epitaxy, and hydrothermal growth. These methods control conditions like temperature, pressure, and solution concentrations to enable crystallization.
Applications
Synthetic crystal growth is widely used to produce materials for electronics, optics, jewelry, and more. Important examples include silicon for computer chips, sapphire for LEDs, and diamonds for gemstones.
Investigating Crystal Defects
Real crystals contain defects – irregularities in their atomic arrangement that affect properties. The field of crystal defects studies these imperfections. Some important concepts include:
Point Defects
Point defects involve individual missing, extra, or replaced atoms in the lattice. Types include vacancies, interstitials, and impurities.
Line Defects
Line defects are 1D disruptions in the lattice such as dislocations and disclinations. Dislocations enable permanent crystal deformation.
Planar Defects
Planar defects are 2D interruptions like grain boundaries between misoriented crystals and twin boundaries across mirror planes.
Volume Defects
Larger 3D defects include voids, inclusions, and other phases like precipitates or bubbles.
Defects can significantly impact physical properties like electrical conductivity, optical transmission, strength, and more. Engineering crystals with controlled defects enables materials innovations.
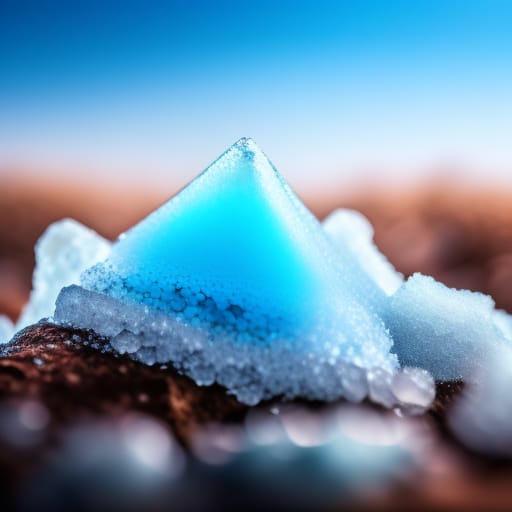
Harnessing Crystal Optics
Crystal optics is the field dealing with how light propagates in crystalline media. Some core concepts include:
Birefringence
Birefringent crystals have a refractive index that depends on light polarization and propagation direction due to their anisotropic structure. This splits light into two rays.
Pleochroism
Pleochroic crystals show variable absorption for different polarizations of light. The crystal appears to change color as it is rotated.
Polarization Rotations
Linear polarized light can have its polarization axis rotated as it passes through some crystals like quartz. Specific rotations depend on crystal symmetry.
Nonlinear Optics
Nonlinear optical crystals like lithium niobate bend light beams, generate harmonic frequencies and enable laser modulation through strong electron interactions with intense illumination.
Crystal optics principles make possible devices like polarizers, wave plates, optical filters, lasers, and electro-optic modulators.
Engineering Crystal Materials
Crystal engineering focuses on rationally designing and synthesizing crystalline materials with desired structures and properties. This relies on:
Crystal Engineering Principles
Key principles from thermodynamics, kinetics, surface science, and quantum mechanics that govern crystallization processes.
Molecular Modeling
Computational modeling of intermolecular interactions to predict crystal packing arrangements.
Crystal Structure Modification
Altering crystal habits, polymorphs, co-crystals, and inclusion compounds to achieve target performance.
Crystallographic Techniques
Advanced X-ray, electron, and neutron diffraction methods to deeply probe engineered crystal structures.
Crystal engineering breakthroughs have enabled advanced pharmaceuticals, organic semiconductors, sensors, energetic materials, and more.
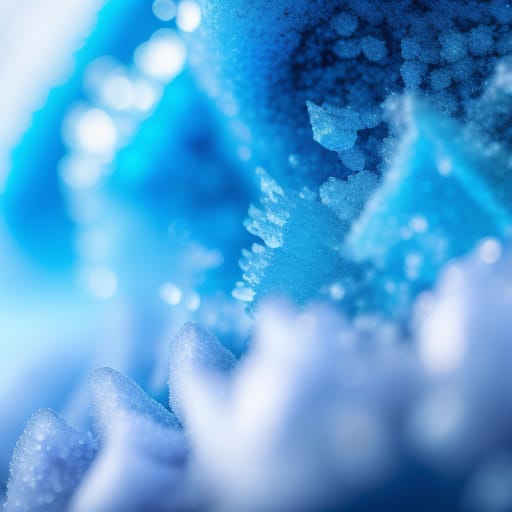
What’s Next for Crystal Science?
As this beginner’s overview illustrates, crystal science is an expansive, interdisciplinary field with many areas to explore. Ongoing research and technology advances are opening new opportunities across crystal science including:
- High-throughput crystallography for accelerated materials discovery
- In situ microscopy to study crystals in dynamic environments
- Advanced computer modeling of crystal properties and processes
- Novel crystal growth techniques like microfluidics and containerless processing
- Nanoscale crystal engineering and assembly
- Biological crystallography innovations
- Crystal-based quantum technologies
The structures and behaviors of crystals will continue providing insights that prove foundational across the physical and life sciences. This introduction to crystal science concepts and applications hopefully sparks your interest to learn more!
Key Resources to Learn More:
- International Union of Crystallography: https://www.iucr.org/
- OpenStax – Introduction to Solid State Chemistry: https://openstax.org/details/books/introductory-chemistry-2e
- Crystallography 365 Blog: https://crystallography365.wordpress.com/
- Crystal Growth Handbook: https://www.springer.com/gp/book/9783527336449
- CrystalMaker Software: https://crystalmaker.com/
- Journal of Applied Crystallography: https://journals.iucr.org/j/journalhomepage.html
Frequently Asked Questions
What is crystallography?
Crystallography is the study of the structure, properties, and arrangement of atoms and molecules in crystalline materials. It involves analyzing crystal structures and crystal symmetries using techniques like X-ray diffraction. Understanding crystallography leads to insights about material properties.
What are some common crystal defects?
Common crystal defects include vacancies (missing atoms), interstitials (extra atoms squeezing into the lattice), dislocations (misaligned planes of atoms), and grain boundaries (misorientations between adjoining crystals). Defects disrupt the periodic crystal lattice and can strongly influence properties.
How are crystals grown?
Some methods to artificially grow crystals include solution crystallization, melt growth, chemical vapor deposition, molecular beam epitaxy, and hydrothermal growth. These techniques rely on slowly crystallizing atoms, molecules, or ions out of liquids, gases, or melts by controlling conditions like temperature, pressure, and concentration.
What is crystal optics?
Crystal optics describes the behavior of light as it propagates through crystalline materials. Key phenomena include birefringence (splitting light beams) due to anisotropic crystal structure and nonlinear optics (frequency doubling, modulation) due to strong light-matter interactions. Crystal optics enables devices like lasers and modulators.
How is crystal engineering used?
Crystal engineering focuses on designing crystalline materials with desired properties by controlling composition, structure, defects, and interfaces at the molecular level. It combines modeling, crystallographic analysis, growth techniques, and nanofabrication. Applications include pharmaceuticals, semiconductors, and sensors.